One cold and overcast November morning, I took my microphones out to Singing Beach, in Manchester, Massachusetts, hoping to record its famous singing sands. Unfortunately, it had rained a few hours before I arrived, leaving the sand too damp to sing.
But, as luck would have it, there was a heavy ocean swell that day: 4- and 5-foot breakers were rolling onto the beach, filling the air with a marvelous and surprisingly steady roar of sound. I stood on shore in dry sand, pointing my shotgun mic towards the area some 25 yards offshore where the waves were breaking.
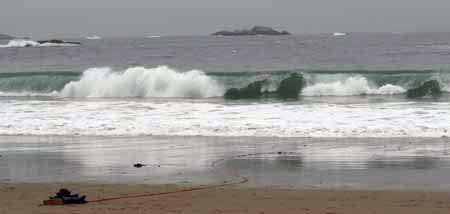
{the red cable snaking out into the surf is for a hydrophone used in a different recording}
Listen to the magnificent slowly rising pitch of the “noise” that follows each breaker’s crash, as the tumbling water rolls ashore, breaking into ever-smaller wavelets, ripples, and popping bubbles of foam. It’s a subtle sound, easily missed during a brisk walk along the beach. But stand still, close your eyes, and face out towards the surf and let your ears alight on the enveloping field of sound that washes over you, and you will hear it, too.
It’s a gorgeously rich natural sound, whose physical origin is surprisingly complex (see the Tech Note, below). For us, standing alone on the keen edge of the shore, it’s enough to turn our eyes and ears oceanward, as each wave announces the end of its journey that began days before, unseen, somewhere in the mid-Atlantic. See the falling, curling wall; feel the thump underfoot and the spitting spray; hear the crash of sound and the rising surge of pitch as foam cascades up the shore: In a thousand voices the wave shouts out its name one final time: I was here! Hear me! Hear me!
Singing Beach lived up to its name today.
Technical note
Here’s how I begin to make sense of the physics of this sound. Upon breaking, a wave disintegrates into a froth of millions of air bubbles trapped in seawater. Within a fraction of second each bubble ruptures, sending out a broad-spectrum pulse of acoustic energy into the air. But upon breaking in this turbulent foam, each bubble in turn spawns a host of smaller ones, which likewise burst into ever-smaller bubbles. So the average size of bubbles tends to decrease as the wave-foam rolls ashore. Now, the smaller bubbles tend to generate more energy at higher frequencies (higher pitch) than do the larger ones, in the same way that a short violin string vibrates at a higher pitch than a long one (all other conditions being equal). The result is a delicious broadband whooosh of sound whose predominant frequency slides upward as the foam comes ashore.
But that’s not all. Because the noise source (the foam) is distributed over a wide area (the foam extends from the wet sand at your feet all the way out to where the waves first break, as well as across the width of the entire beach), each little bubble-pulse of sound arrives at your ears at a slightly different time, resulting in a wildly complex pattern of constructive and destructive interference around your ears. Moreover, some of the foam-sound travels directly to your ears, while some is reflected off the wet sand. This travel-time difference produces a “comb” filter effect, accentuating some frequencies at the expense of others. As the foam moves ashore, this time-delay decreases, causing the overall pitch to slide upward.
(You can hear comb-filtering at work the next time you’re outdoors as a plane flies overhead. If you lean your head down to the ground (or simply squat slightly) you should hear the average pitch of the plane’s noise rise as your ears moves closer to the ground. By moving your ears closer to the ground, you decrease the time delay between the sound coming directly from the plane and that reflected off the ground.)
Finally, remember that all these minute sound sources are in motion relative to you, the listener. At first, when the wave breaks, the bubbles are moving towards you quite rapidly. But as the water rolls up the shore, its energy dissipates and its (and the bubbles’) forward movement slows. In the same way that the pitch of a train whistle decreases as the train flies past, we’d expect the pitch of the foam to decrease as the waves’ forward movement slows. Although this Doppler effect should lower the pitch, the relative velocity of the foam is so low that the effect is probably negligible. So of the two competing effects comb-filter and Doppler the former wins: the pitch rises.
P.S. The moving pitches in this recording are 100% natural. No variable bandpass filters were used. It’s the real deal.